Decoding LNP: mRNA Encapsulation Process and Applications! The 5th issue of the series was launched
In the 60s of the 20th century, it was discovered that lipids can spontaneously form closed lipid bilayer vesicles in water, and the term “liposome” was born. With the beginning of the era of nanoscience and nanotechnology, the term “lipid nanoparticle (LNP)” only began to be used in the early 90s of the 20th century. The brains behind mRNA vaccines against Covid-19 are LNPs that encapsulate mRNA and safely and effectively deliver it into the body’s cells.
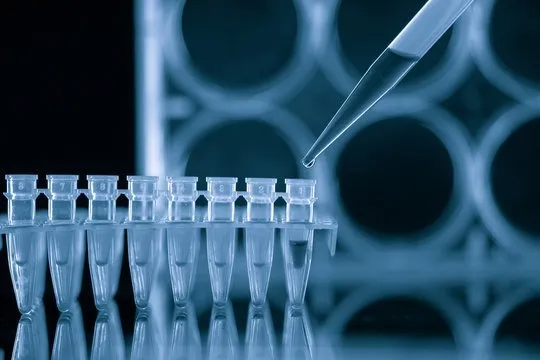
Encapsulating mRNA in LNPs and then entering the human body not only has low toxicity, low immunogenicity, excellent kinetic stability, and robust structure, but also protects mRNA from nuclease destruction in the systemic circulation, and efficiently delivers mRNA into cells through a fusion mechanism with the lipid bilayer of early endosomes. It is the only mRNA delivery technology approved by the FDA and has been used in hundreds of millions of doses of mRNA vaccines around the world, and its safety and efficacy have been verified in this global new crown epidemic.
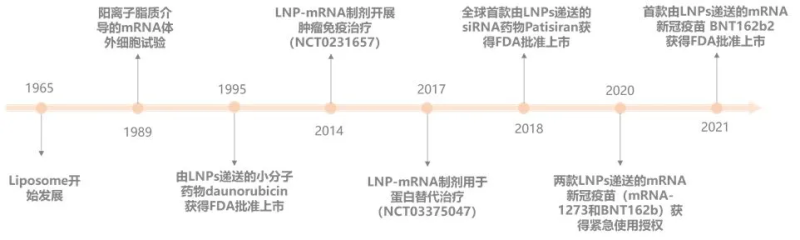
The development history of LNP technology
LNP type
According to the different structure and drug loading mechanism of lipid nanoparticles, LNPs can be divided into: cationic LNPs, Lipid polymer hybrid NPs (LPHNPs), calcium phosphate (CaP) LNPs (LCPs) and ionizable LNPs[1]。
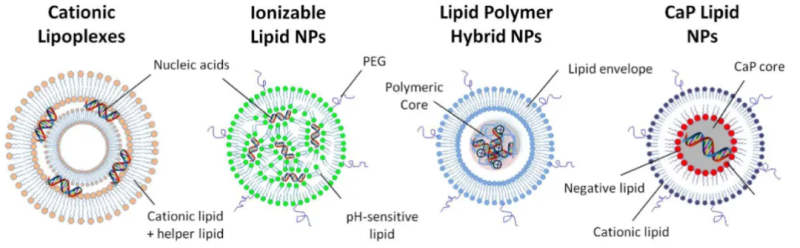
01 Cationic lipid nanoparticles (Cationic LNPs).
Cationic LNPs were one of the first synthetic materials for gene delivery[2] and consist of cationic lipids, which have the ability to strongly bind and concentrate negatively charged nucleic acids into stable nanoparticles, known as LPX (lipoplexes), and neutral accessory lipids. This LPX is very effective in vitro but is unstable in aqueous solution and has limited efficiency in delivery in vivo due to their short half-life in the body circulation.
02 Lipid Polymer Hybrid NPsLPHNPs
LPHNPs are made up of nucleic acids concentrated with lipid and cationic polymers. This system combines the advantages of cationic lipids and polymers in gene delivery. Polycations reduce the amount of cationic lipids used and improve the nuclear delivery of DNA[3]. The lipid layer of the outer layer provides additional protection for nucleic acids and can be modified with different ligands and devices to improve pharmacokinetics and cell-specific targeting[1].
03 Lipid calcium phosphate NPs (LCP NPs).
LCP NP is the most versatile platform for the delivery of a wide range of nucleic acids. The nucleic acids combine with calcium phosphate (CaP) to form a core, which is then coated with different lipids to form a core-shell structure known as LCP-1. LCP nanoparticles are characterized by a small diameter, less than 50nm, which facilitates hepatocyte delivery through the sinusoidal window pores of the liver[4].
04 Ionizable LNPs
Ionizable lipid LNPs are typically made up of four components: ionizable lipids, phospholipids, cholesterol, and polyethylene glycol (PEG) lipids. Ionizable lipids are the most critical excipients in LNP delivery systems, and are particularly important in determining mRNA delivery and transfection efficiency, with ionizable cationic lipids having a pKa of less than 7 and therefore positively charged in acidic media and electrically neutral under physiological conditions. It can interact with nucleic acids and wrap them under acidic conditions (pH=4), and then change the buffer system to make pH=7.4 to form neutral LNPs, which are not only suitable for systemic drug delivery, but also avoid the disadvantages of permanently positively charged LNPs [5]; PEG lipids have a significant effect on the regulation of particle size and zeta potential, promote particle stabilization by reducing particle aggregation, and can also prevent serum proteins from binding to nanoparticles, reducing the clearance of nanoparticles in the systemic circulation, thereby prolonging the systemic circulation time. Phospholipids are generally neutral, providing bilayer structural stability for LNPs, while improving the permeability of cell membranes and promoting cell uptake and endosomal escape. Cholesterol improves particle stability by regulating membrane integrity and rigidity [6].
Ionizable lipid LNPs have two additional advantages, first, they bind to circulating endogenous proteins, such as apolipoprotein E (ApoE), which efficiently mediates the delivery of ionizable lipid LNPs to hepatocytes via the low-density lipoprotein (LDL) receptor pathway. Second, in the acidic pH environment of the endosomes, these lipids acquire a positive charge and can bind to the negatively charged lipids on the endosome membrane to form a non-bilayer structure, inducing the rupture of the endosomes, thereby causing the escape of the endosomes. Due to this potent endosomal escape process, the use of ionizable lipids for siRNA and mRNA delivery yields much greater gene silencing and expression in vitro than cationic lipids. These advantages have made ionizable lipids a key ingredient in some of the mRNA vaccines currently on the market, such as Moderna’s Covid-19 mRNA vaccine, which uses the self-developed ionizable lipid SM-102, and Pfizer and BioNTech licensed an ionizable lipid called ALC-0315 from Acuitas. In the following, we will focus on the preparation process of ionizable lipids.
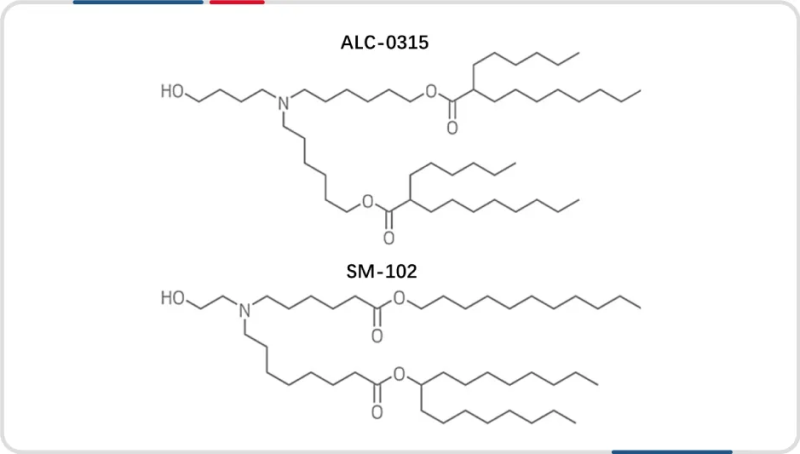
Two ionizable lipid formulas for the application and commercialization of mRNA vaccines
Key quality parameters of LNPs
Key quality indicators during LNP production include particle size, polydispersity index (PDI), encapsulation efficiency, zeta potential, mRNA integrity of the formulation, and content of each component in the delivery system.
01 Particle size and PDI of LNPs
The average particle size and particle size distribution PDI of LNPs are important factors in the quality of LNPs and suitability for various applications, and are often characterized by dynamic light scattering (DLS). Studies have shown that LNPs with different particle sizes are expressed differently in vivo: the smaller the LNP size in the case of intramuscular administration, the more likely it is to migrate to the liver to show liver targeting, while the larger particle size is mostly left at the injection site [7]. In addition, the size of LNPs may affect the internalization, biodistribution, degradation, and clearance of LNPs, and different applications may require different particle sizes[8]. The optimal particle size range for LNPs is generally considered to be 20−200 nm with a PDI of less than 0.2.
02 Encapsulation rate of LNPs
Encapsulation efficiency is a very critical indicator of mRNA-LNP, which indicates the proportion of RNA encapsulated in lipid nanoparticles to total RNA, which is usually characterized by fluorescent dye method, which mainly affects the delivery efficiency, stability, and safety of drugs. High encapsulation efficiency LNPs can ensure that more drug molecules are effectively delivered to target cells, improving treatment efficacy; More stable in the in vitro and in vivo environment, resulting in less loss of the drug during delivery; It can also reduce the exposure of drug molecules, reducing the risk of immune reactions and side effects. Typically the default qualified LNP encapsulation rate is >90% [9].
03 The mRNA integrity of the LNP preparation
The stability and integrity of mRNA is crucial for the performance of its function, LNP, as the carrier of mRNA, encapsulates mRNA in it through specific technical means to protect mRNA from degradation by enzymes in the body, and at the same time helps mRNA to enter cells efficiently, if mRNA is degraded in LNP, it will affect its ability to code for proteins, and then affect the immune effect of vaccines. Therefore, the mRNA integrity parameters in LNPs are crucial in the quality control system, and the requirements for mRNA integrity of LNP preparations vary from industry to industry
04 Zeta potential of LNP
The LNP zeta potential, which is the electrostatic potential surrounding the LNP, is a good indicator of the stability of the nanoparticle suspension and its potential side effects, and can be measured by a variety of techniques such as electrophoretic light scattering (ELS), microelectrophoresis, and more. Zeta potential affects the colloidal stability, aggregation behavior, flocculation rate, and interaction with biofilms of LNPs, among other things. The higher the absolute value of the zeta potential, the higher the electrostatic repulsion and better stability of the LNP, while the neutral zeta potential leads to LNP agglomeration and potential agglomeration.
05 Identification and content determination of lipid components of LNP
The USP draft Procedures for the Quality Analysis of mRNA Vaccines states that the LNP lipid fraction and content of mRNA vaccines need to be determined. In order to meet the needs of method development and detection of mRNA-LNP, Hanhai New Enzyme established an applicable ELSD-HPLC analysis method, and the four LNP components were strongly retained, achieving complete separation and successful identification of lipid components.
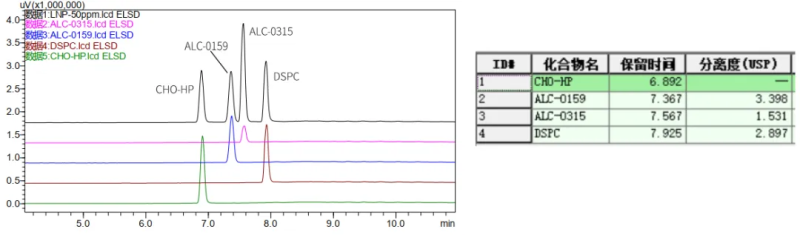
A certain concentration of standard solution was injected into 10ul and 5ul respectively, a standard curve was established, and the unknown sample was diluted 10-fold and then injected for analysis, and the quantitative results were as follows:
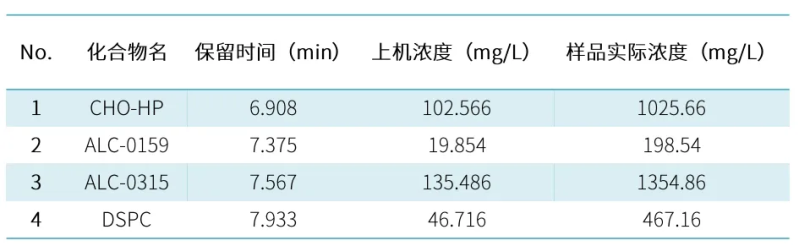
Optimization idea of LNP preparation by microfluidic process
The commonly used preparation methods of lipid nanoparticles mainly include thin film hydration method, extrusion method, homogenization method, macrofluidics/T junctions, etc. However, the average particle size of LNPs produced by this method is relatively large, and the wrapping rate is low. At present, the most widely used is microfluidic hybrid technology, which is relatively simple and fast, mild and easy to scale-up.
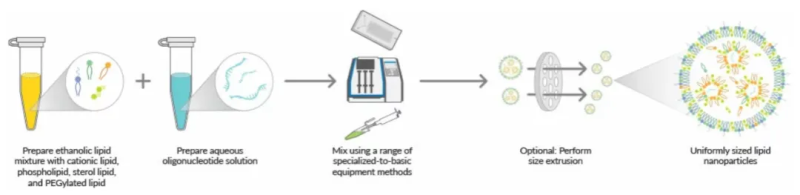
LNP microfluidic control backup process
The optimization of the preparation process of lipid nanoparticles is a complex process with multiple factors and parameters, which involves many aspects such as material selection, process parameter adjustment, and final product performance evaluation.
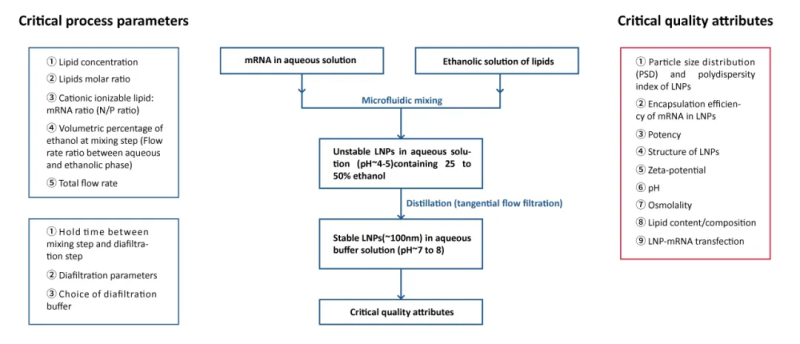
LNP preparation process and process quality parameters
During the preparation of lipid nanoparticles, the molar ratio of each lipid component determines the composition of LNPs and affects their particle size, PDI, and efficacy. Due to the ability of PEG-lipids to inhibit the binding of ApoE to LNPs, excess PEG-lipids may adversely affect cellular uptake and transfection of LNPs. LNPs containing less PEG-lipids enable more efficient nucleic acid delivery. The two earliest marketed mRNA vaccines had a PEG-lipid ratio of 1.5% and 1.6%, respectively.

Mole ratio and nitrogen to phosphorus ratio of each component of some marketed vaccines
Studies have been shown [10][11] that the flow rate ratio of the aqueous and lipid phases and the total flow velocity in the aqueous and lipid phases significantly affect the particle size of LNPs. On this basis, Hanhai New Enzyme further studied the factors affecting the particle size of LNPs. The experimental results show that PEG%, TFR (Total flow velocity) and FRR (Flow rate ratio) are inversely proportional to particle size. The aqueous salt concentration is proportional to the particle size; N:P and aqueous pH have little effect on particle size.

Effect of different process parameters on the physicochemical properties of LNP
Hzymes has explored and optimized the downstream purification process of LNP, mainly for the inlet flow rate, transmembrane pressure difference TMP (Trans-Membrane Pressure Drop), membrane pore size, membrane area, membrane material, hollow fiber and flat membrane cassette, the process time can be controlled at about 3.5h, the stability of mRNA-LNP preparations at -80°C can reach more than 18 months, and it can be frozen-thawed10 does not affect physical and chemical indicators.
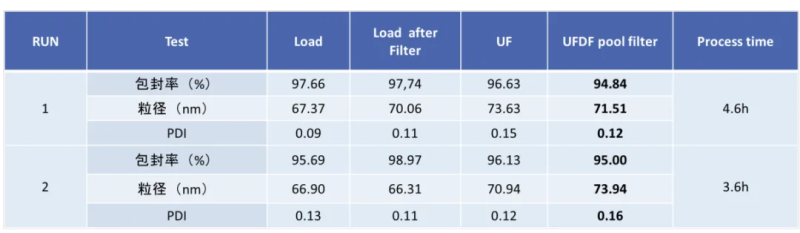
Hzyme has advanced technology and rich experience in mRNA production and LNP preparation, and adopts simple, fast, high-stability, high-quality microfluidic technology for the preparation of mRNA-LNP, which can provide customized services for mRNA and mRNA-LNP with different specifications, and can customize LNPs with different particle sizes according to customer needs, and provide mRNA-LNP encapsulation efficiency, particle size, PDI, and Zeta potential and other related quality control testing services.
Based on the cumbersome preparation process of traditional LNPs, various equipment is required, resulting in high application costs and greatly limited throughput in early research or scientific research stages. In order to help customers quickly validate targets in the early research or scientific research stage, Hzymes Biotech
Hzyme provides:
A full set of mRNA vaccine & drug-related high-performance enzyme raw materials, chemical substrates, IVT kits and quality control kits, with independent plasmid preparation, IVT process development, mRNA purification, LNP encapsulation and related quality inspection platforms, can provide customers with a full set of solutions based on raw materials, comprehensive process development services, and help customers quickly promote the development of new drugs. For early-stage customers, we can provide CRO services, proof-of-concept services, third-party quality inspection services, and various finished mRNA stock solution products, including reporter genes, target proteins, gene editing proteins, and antigen proteins.
References
[1] Khalil IA, Younis MA, Kimura S, Harashima H. Lipid Nanoparticles for Cell-Specific in Vivo Targeted Delivery of Nucleic Acids. Biol Pharm Bull. 2020; 43(4):584-595.
[2] Malone RW, Felgner PL, Verma IM. Cationic liposome-mediated RNA transfection. Proc Natl Acad Sci U S A. 1989 Aug; 86(16):6077-81.
[3] Masuda T, Akita H, Harashima H. Evaluation of nuclear transfer and transcription of plasmid DNA condensed with protamine by microinjection: the use of a nuclear transfer score. FEBS Lett. 2005 Apr 11; 579(10):2143-8.
[4] Satterlee AB, Huang L. Current and Future Theranostic Applications of the Lipid-Calcium-Phosphate Nanoparticle Platform. Theranostics. 2016 Apr 27; 6(7):918-29.
[5] Tam YY, Chen S, Cullis PR. Advances in Lipid Nanoparticles for siRNA Delivery. Pharmaceutics. 2013 Sep 18; 5(3):498-507.
[6] Li X, Qi J, Wang J, Hu W, Zhou W, Wang Y, Li T. Nanoparticle technology for mRNA: Delivery strategy, clinical application and developmental landscape. Theranostics. 2024 Jan 1; 14(2):738-760.
[7] Di J, Du Z, Wu K, Jin S, Wang X, Li T, Xu Y. Biodistribution and Non-linear Gene Expression of mRNA LNPs Affected by Delivery Route and Particle Size. Pharm Res. 2022 Jan; 39(1):105-114.
[8] Eygeris Y, Gupta M, Kim J, Sahay G. Chemistry of Lipid Nanoparticles for RNA Delivery. Acc Chem Res. 2022 Jan 4; 55(1):2-12.
[9] Muramatsu H, Lam K, Bajusz C, Laczkó D, Karikó K, Schreiner P, Martin A, Lutwyche P, Heyes J, Pardi N. Lyophilization provides long-term stability for a lipid nanoparticle-formulated, nucleoside-modified mRNA vaccine. Mol Ther. 2022 May 4; 30(5):1941-1951.
[10] Roces CB, Lou G, Jain N, Abraham S, Thomas A, Halbert GW, Perrie Y. Manufacturing Considerations for the Development of Lipid Nanoparticles Using Microfluidics. Pharmaceutics. 2020 Nov 15; 12(11):1095.
[11] Malburet C, Leclercq L, Cotte JF, Thiebaud J, Bazin E, Garinot M, Cottet H. Taylor Dispersion Analysis to support lipid-nanoparticle formulations for mRNA vaccines. Gene Ther. 2023 May; 30(5):421-428.
Please first Loginlater ~